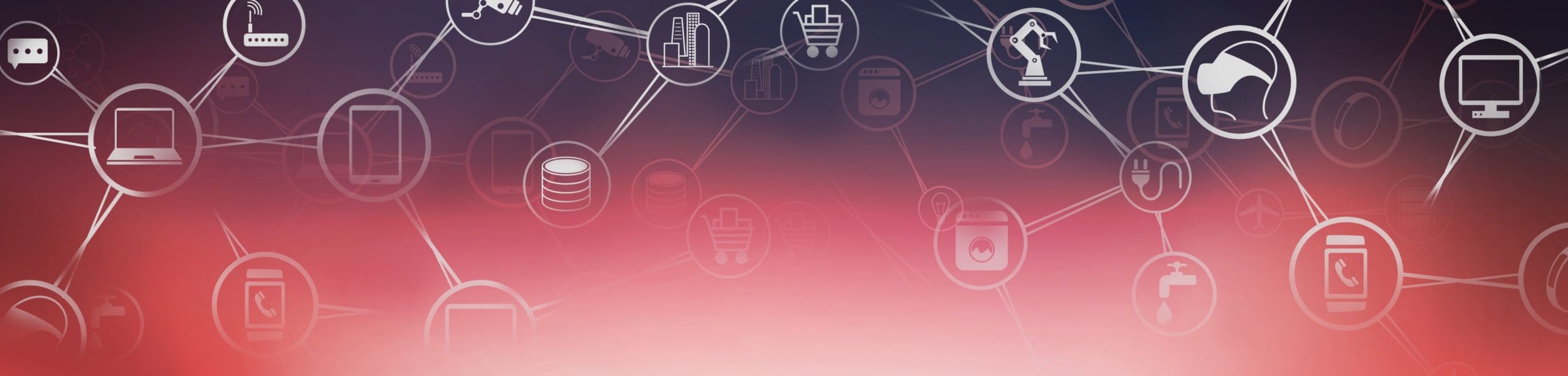
Over the past five decades, the engineering education community has explored and established effective techniques to teach design. In the emerging world of transformational technologies of IoT, sustainable energy, machine intelligence, etc., the question of whether the existing successes can readily adapt to these emerging realities is triggering some vigorous discussions among faculty.
Challenges for Modern Design Education
My company, Quanser, has been working with various universities to update the technological and pedagogical framework to bolster current practice to respond to these challenges. Some key factors that our work is addressing include:- Technological complexity: The interplay between the digital and the traditional analog world has produced a profound shift in the way engineers in industry design.
- System-level complexity: Emerging engineering systems are often characterized by networks of “things” each with its own behavior and a dynamic interaction the network’s collective behavior.
- Socio-economic complexity: This includes entrepreneurship or industry-readiness and the “flip side” concerns of engineering ethics in a world of machine learning and artificial intelligence.
- Institutional complexity: The traditional course/class structural constraints opportunities alternate structures such as flipped classroom on-line learning, micro-learning/badging, etc.
A Taxonomy of Design
Design itself is an overloaded word. There is, for many, a de facto definition as it is addressed in most programs. It will be some combination of a thought process that encompasses ideation, evaluation of alternatives, then prototype and testing. Additionally, key tools skills involving software and perhaps fabrication are also common. But in the face of the above complexities, it may be helpful to dissect the concept of engineering design in the context of modern systems. For the Quanser approach, we suggest a progression of design complexities:- Instinct-based Design (Intuitive Systems): Conceiving and building things based on common experiences for most students. The challenges to channel those instincts in a cohesive, purposeful way. This is typical of the freshman design project.
- Model-supported Design (Described Systems): Applying engineering mathematics and science to accelerate and optimize design systems with more intricate dynamics. In some sense, this is what many faculties aim to teach as they help students reconcile the techniques of the engineering science courses with the design courses.
- Validated Design (Instrumented Systems): To harmonize the empirical or measured view of systems with the modeled view to effect more accurate and robust designs.
- Complex System Design (Connected Systems): To shift the view from the device (or the proverbial “widget”) to a network and to consider the interdynamics and the collective function.
A “Rhythm” of Authentic Design Education
The approach under construction suggests a certain sequence or “rhythm” of design education:- Motivation (First Contact): “Fall in love” with the application. If it is robotics, then have students engage in an exciting way with a real robot or stress the societal importance of the field.
- Foundation 1 (Technical skills primitives): The necessary technical skills needed to be proficient in designing systems of any complexity in the target field. Power, vision, microcontrollers, control, etc. would be examples for robotics. But these would be packaged in usefully abstracted, compact learning units, often hands-on so that students can efficiently develop a broad range of skills with practical levels of depth in a “sprint’ fashion.
- Design Project 1 (Guided design): A compact, strongly scaffolded design project where students focus on the modification and creation of a particular functional dimension in a more complex system while the other subsystems are provided. This balances design thinking with meaningful complexity for the project, and it connects the skills primitives more explicitly with system function.
- Foundation 2 (Vertical skills): A subsequent sprint of integrative topics and skills that map the foundational tools to the needs of the application. For example, if a student is designing a robot, higher level concerns of machine learning, advanced control, etc. may fall in. Or if the context is more entrepreneurial, then Design for Manufacture could provide the framework. Additionally, a structured treatment of socio-economic and ethics topics can complement the technical progression.
- Design Project 2 (Open design): The more conventional, large design project (e.g., capstone) concludes the sequence. The hypothesis here is that the above progression or “rhythm” that balances motivation, with horizontal and vertical skills can better prepare students for a more comprehensive experience and ambitious design results in the concluding project.